Pocillopora acuta Lamarck, 1816
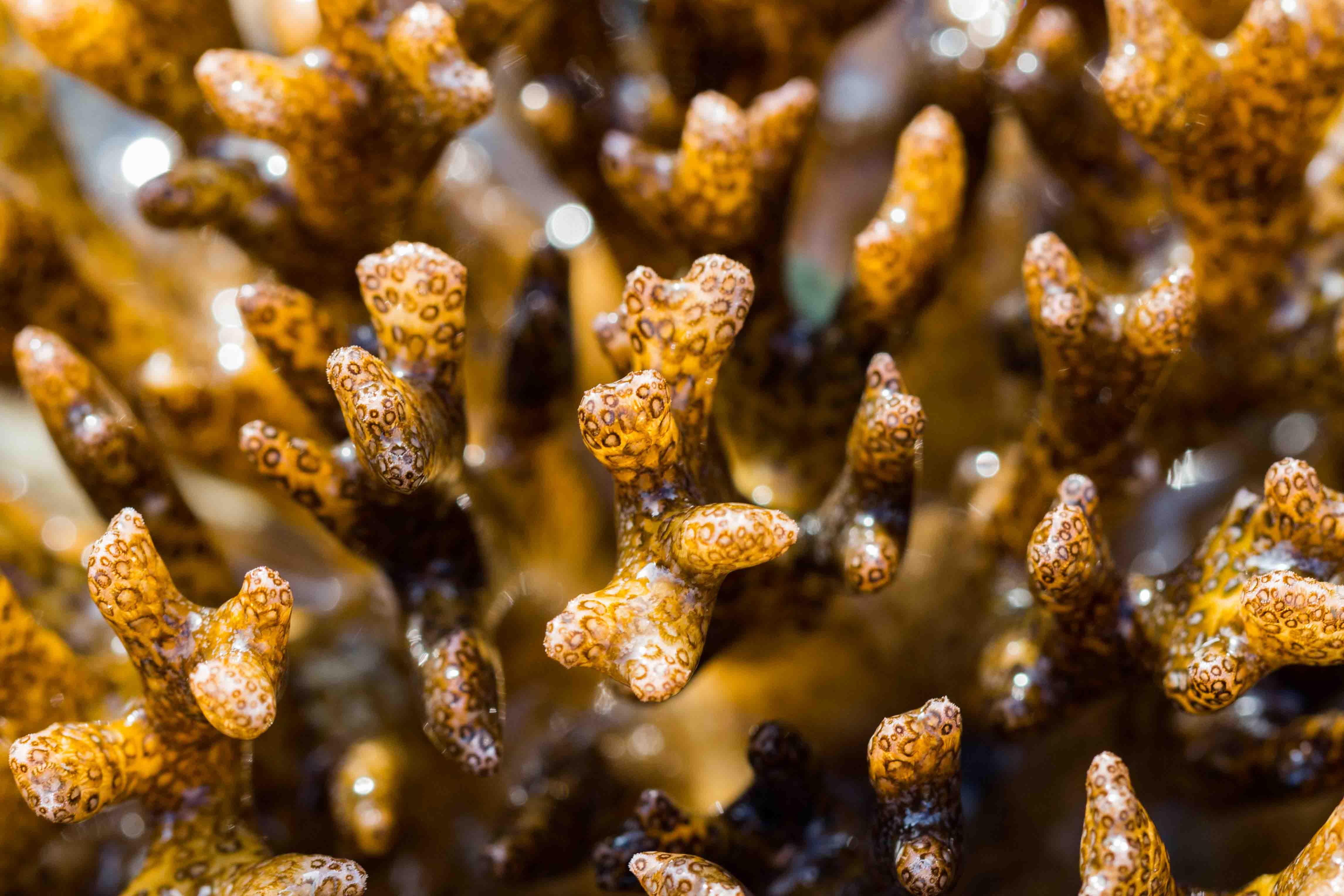 |
Pocillopora acuta exposed in the intertidal Pulau Hantu, Singapore. (Image by: Goh Yi Le) |
Overview
Pocillopora acuta is a species of colonial hard coral consisting of many individual animals known as polyps that deposit a calcium carbonate skeleton. They have symbiotic algae (or zooxanthellae) from the genus Symbiodinium within cells in the inner tissue layer of the cnidarian animal host [1]. The algae in their tissue is also responsible for giving the corals their unique and beautiful colouration. This symbiosis provides food to the corals and is crucial to the ability of corals to deposit their massive calcium carbonate skeletons that form the structure of coral reefs [2]. Corals from the genus Pocillopora including Pocillopora acuta are widely used models in ecological [3], experimental studies to investigate the effect of climate change (rising ocean temperature) on corals [4], and even the effectiveness of coral transplantation to restore coral reefs [5]! Watch the video below to understand more about corals and coral reefs!
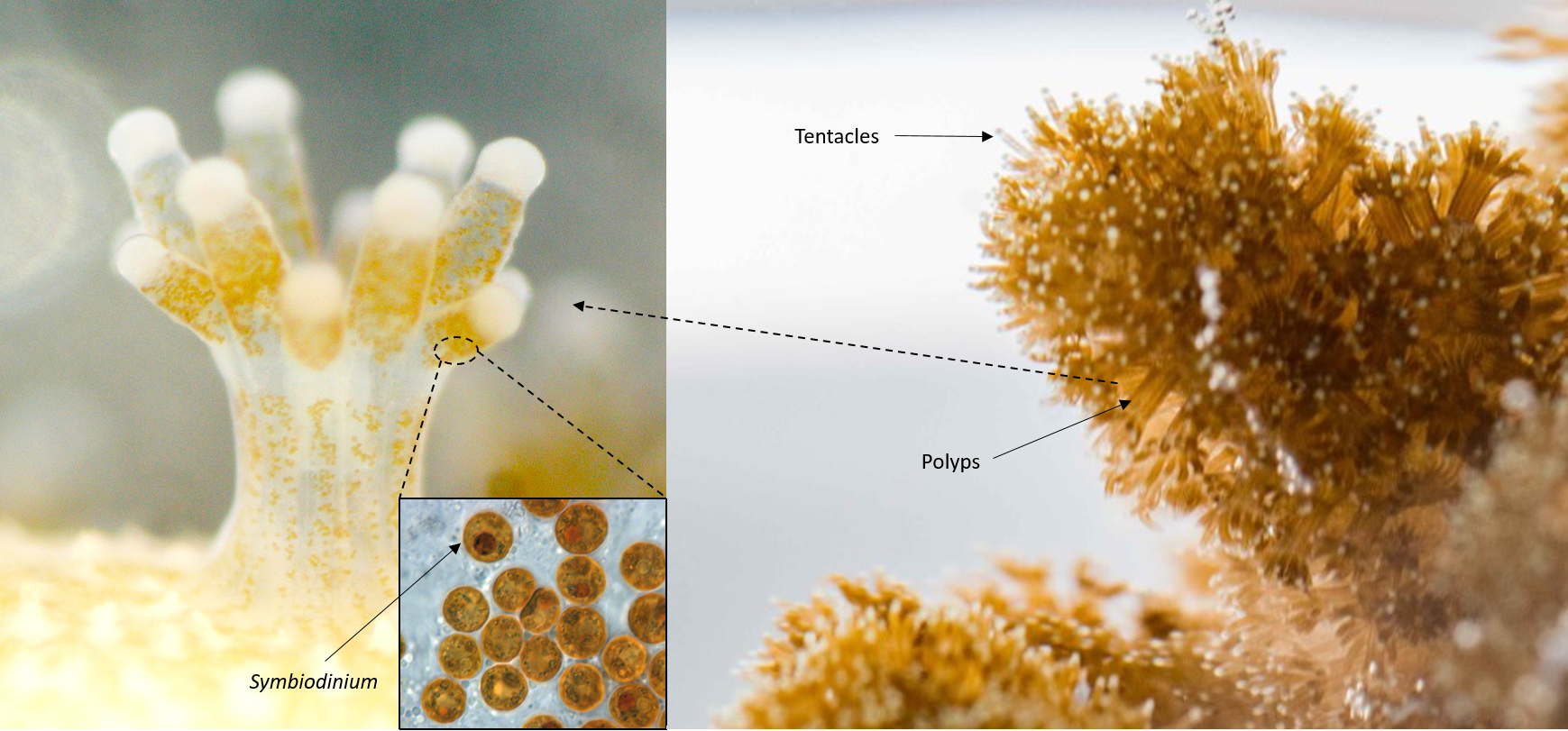 |
Collage of photos from different sources showing coral polyps with symbiotic alage from the genus Symbiodinium within them and Pocillopora acuta polyps with tentacles extended (source: Noriyuki Satoh, Okinawa Institute of Science and Technology Graduate university; Allisonmlewis, Symbiodinum wikipedia; Goh Yi Le) |
Distribution and habitats
Pocillopora acuta is widely distributed from the Central Pacific to the Indian ocean, including Singapore [6, 7]. It is common on Singapore's reefs [8], intertidal areas of southern shores, and artificial substrates such as seawalls [9] and pontoons [10].
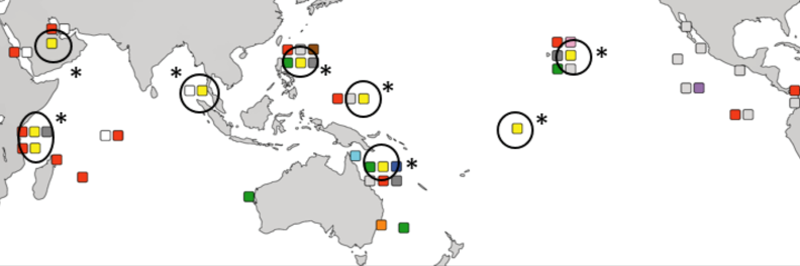 |
Global geographical distribution of Pocillopora acuta with yellow squares and black circles with astericks indicating locality. (Source: Schmidt-Roach et al., 2014) |
Biology and ecology
Feeding
The autotrophic zooxanthellae photosynthesizes and supplies photosynthetically fixed carbon to the heterotrophic Cnidarian host, while the host provides nutrients such as inorganic nitrogen, phosphorous and shelter in a high light environment [1]. The cnidarian hosts are capable of feeding on a range of food sources including dissolved organic matter, suspended particulate matter, and zooplankton [11, 12, 13]. The main feeding method utilized by corals is via the use of tentacles [1]. The nematocysts (needle like sting) in the cnidocytes (sting carrying cells) of the coral tentacles immobilize the prey, while the prey is deposited directly into the mouth or onto the mucus around the oral disk by tentacular contraction and folding towards the polyp [14].
Coral bleaching and mass bleaching events
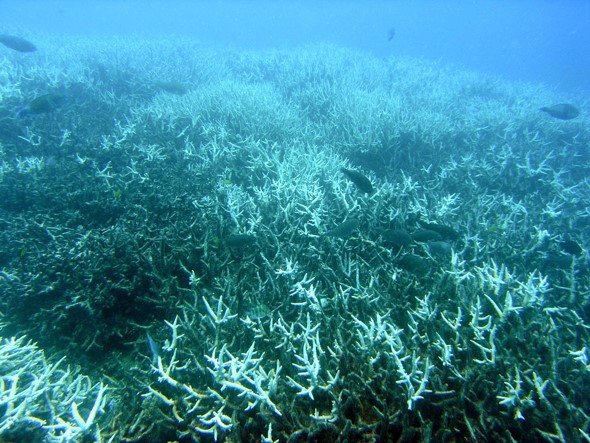 |
Bleached branching coral (Acropora sp.) at Heron Island, Great Barrier Reef. (Photo by: J. Roff) |
Coral bleaching is when coral polyps release the symbiotic algae that live inside their tissue or the photosynthetic pigments (like chlorophyll) within the algae, making the corals paler, lighter, and sometimes even completely white [15]. This can be due to many different disturbances or factors working together and/or independently. Bleaching is primarily thought to be due to an increase in water temperature (exceeding local summer maximum by 0.5-1.5°C) [16] and increased solar irradiance [15]. Other causes include reduced water temperature, high sedimentation, bacterial infections, salinity changes and even pollutants [15]. While corals can continue to survive, prolonged exposure to these conditions might results in the coral polyps dying and decaying, leaving their calcium carbonate skeletons behind. When this occurs over large geographic scales, it is known as mass bleaching events. 3 major pantropical mass bleaching events are thought to have occured in 1998, 2010 and 2015 [17]. Another recent mass-bleaching in 2017 affected nearly two-thirds of the Great Barrier Reef [18].
Microhabitat for other animals
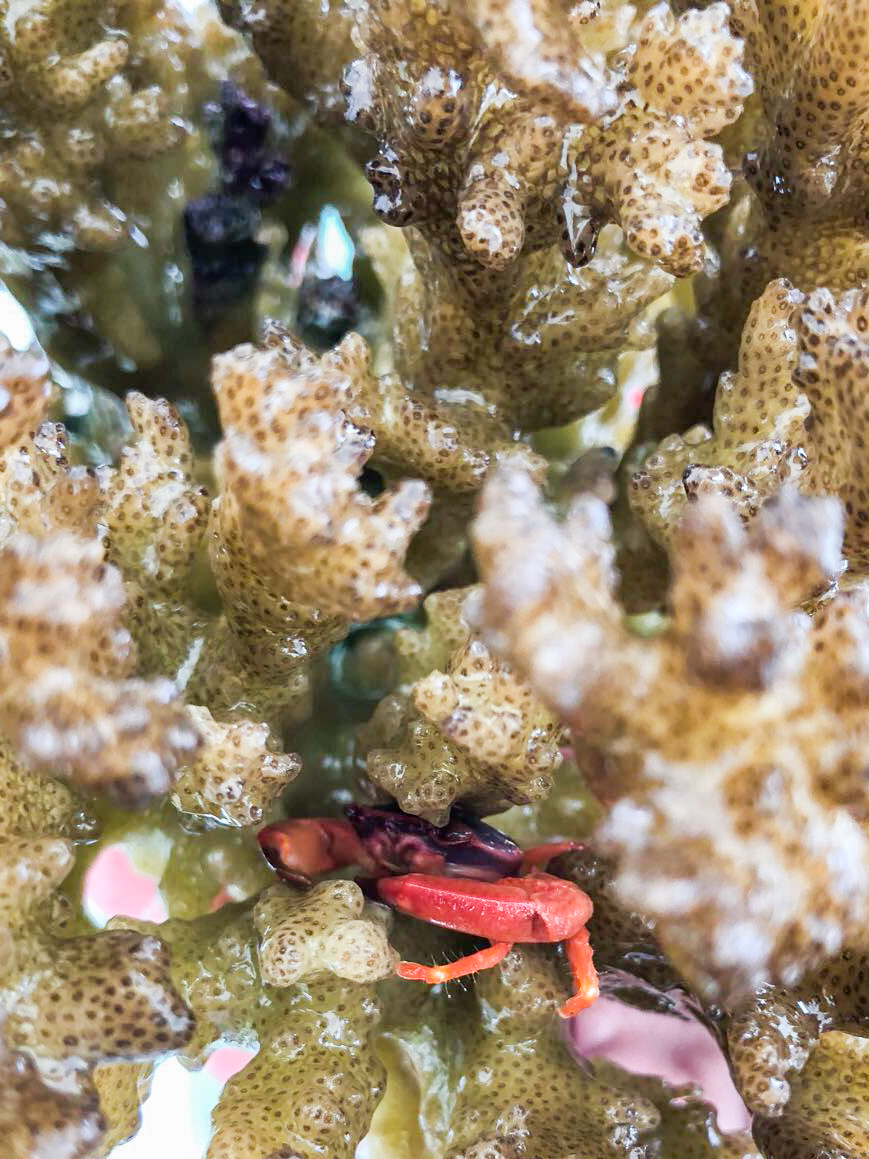 |
Photo of the red coral crab (Trapezia cymodoce) living within the branches of Pocillopora acuta (Photo by: Lim Zi Wei) |
Pocillopora corals are known to be home to small animals like crabs and shrimps living within their branches. The red coral crab (Trapezia cymodoce) receive protection from predators as well as food source (coral mucous) while it helps clean the coral by removing excess sediment [19].
Reproduction
Pocillopora acuta are hermaphroditic [20], meaning they possess both male and female sexual organs. It is able to reproduce using sexual spawning or asexual brooding, budding and even fragmentation. Brooding is where eggs develop to free swimming larvae known as planula in gastrovascular cavity of parent polyp, while sexual spawning is where eggs and sperms are released into the water column where fertilization and development occurs [21]. It is thought that a mixed mode of reproduction consisting of asexual brooding and sexual spawning is a synapomorphic (or shared novel character) for the monophyletic group containing P. damicornis, P. aliciae, P. acuta which originated from a shift in its reproductive strategy (i.e. from ancestral spawning to mixed mode of asexual brooding and sexual spawning) [6]. It is known to brood monthly in Singapore, just before or after the new moon [22].
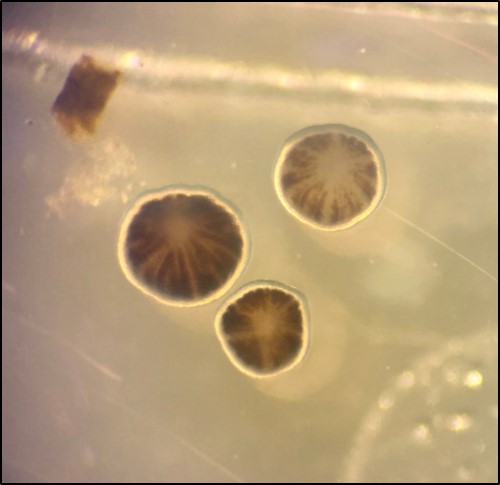 |
Photo showing planulae released from Pocillopora acuta coral just before the new moon. (Photo by: Lim Zi Wei) |
Significance
Ecosystem services and function
Corals like Pocillopora acuta make up coral reef systems in the central Pacific to the Indian Ocean [7], but why should we care what happens to coral reefs? Watch the video below to find out!
Coral reefs are incredibly important ecosystems, providing vital ecosystem services and functions estimated to be worth up to 2,129,122 $/hectare/year [23]. This includes fisheries where coral reefs serve as habitat and nuseries for many commercial species of fish, novel biochemical compounds such as anti-cancer drugs, coastal protection against storms and erosion, tourism, and materials such as sand [24]. Watch the video to see how Parrotfish turn corals into the sand that form our beaches and use for building material!
Threats
Climate change
With the onset of climate change, scientists predict that coral reefs are expected to experience not only more frequent but also more intense bleaching events [17]. Corals not only have to face the effects of rising average ocean temperature but increasing ocean acification due to rise in carbon dioxide in the atmosphere. The ability of corals to adapt and or acclimitize to these changes are thought to be crucial for their survival [25].
Blast fishing
Blast fishing or dynamite fishing is the illegal practice where explosives are used to kill or stun fish while simultaneously
destroying the underlying coral reef habitat which is comprised of corals like Pocillopora acuta that supports the fish. This reduces the structural complexity of the reef or reduces the number of microhabitats for different animals to grow, live and settle among [26].
Coastal modification, land reclamation, and dredging
Land reclamation, coastal modification and the related process of dredging is highly destructive to coral reefs as it may involve the removal of substratum and associated organisms from the seabed and subsequent burial of any survivng organisms by sediment deposition [
27]. This also increases the turbidity in the water due to increased sedimentation, allowing for less sunlight for photosynthesis. Furthermore, corals require energy to remove/reject sediment covering it, leaving less energy available for for growth, reproduction and competition [
28]. Watch the video below to see how corals reject sediments! In Singapore between 1920s and 1990s, there was a pronounced lost of natural coastline dropping from 98% to 40% resulting in the reduction of nearly half the coral reef area (from 32
km2 to 17 km2) and intertidal sand/mudflat area (33 km2 to 8 km2) [
29]. This reduction is projected to continue in the future [
30].
Pollution, eutrophication and phase shifts
Pollution such as sediment and nutrient run off,
eutrophication often lead to the degradation of coral reef ecosystems.
Eutrophication is the enrichment of water bodies with excess nutrients. It leads to enhanced macroalgal growth and when there is unusually low rates rates of herbivory, possibly a phase shift of the coral reef from a coral dominated state to a macroalgal state [
31]. The change is often, but not necessarily always associated with perturbations such as mass coral bleaching and mortality, outbreaks of coral eating species, or even storm damage [
32].
Taxonomy and systematics
Description and morphology
Description is from a study by Poquita-Du et al., 2017 [
7]
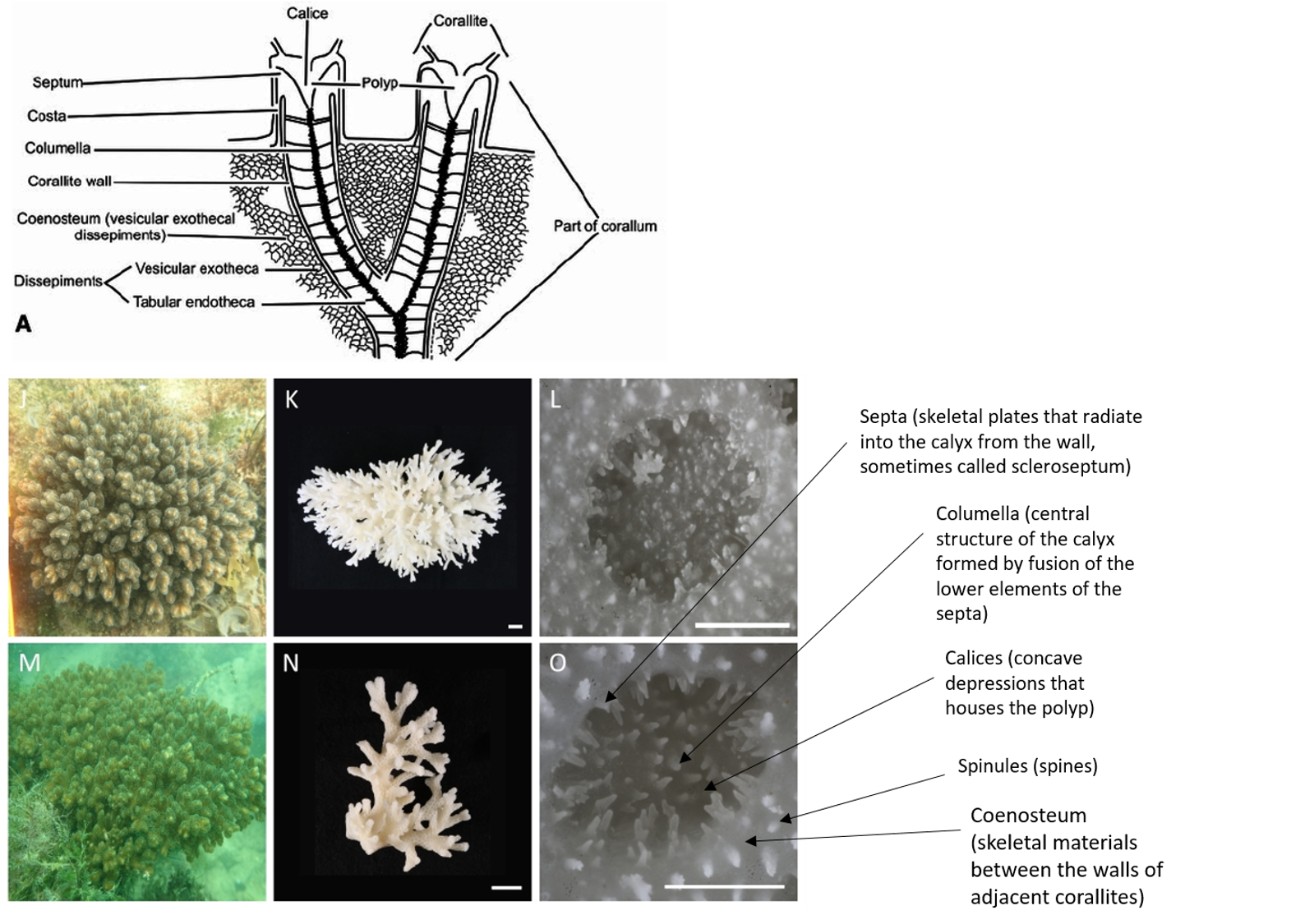 |
Drawing of coral morphology and photos of Pocillopora acuta (Source: drawing by Wells from Treatise on Invertebrate Paleontology; Photos by Poquita-Du et al., 2017) |
Colonial, densely caespitose; branches typically round in cross section, but may become flattened at the tips, which are usually sharply pointed. Branches of colonies in exposed sites thicken and have smaller spacing between branches, while those in sheltered sites are elongate and slender. Calices typically oval, with the small diameter ranging between 0.6 and 0.8 mm, and the large diameter between 1.0 and 1.2 mm. Columellae flat. Septa poorly developed, in two equal cycles; 12 septa per corallite. Coenosteum with fine spinules. Living colony pale-greenish in colour with characteristic darker pigmentation surrounding oral opening of polyps.
Type Information
A type specimen refers to a specimen or a group of specimens that functions as the reference to all defining characteristics of that species, to which a species (scientific) name is officially attached to.
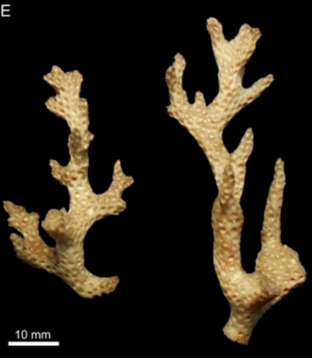 |
Photo showing side view of skeleton of holotype of Pocillopora acuta (photo by: Michel Pichon from Schmidt-Roach et al., 2014 [6]) |
The holotype for Pocillopora acuta resides in the Muséum national d’Histoire naturelle de in Paris, France (MNHN-IK-2010-792). The type locality is the Indian Ocean [6].
Classification
Binomial name: Pocillopora acuta Lamarck, 1816
Order: Scleractinia Family: Pocilloporidae Genus: Pocillopora
|
|
Taxonomic Hierachy
|
|
Kingdom
|
Animalia
|
Phylum
|
Cnidaria
|
Class
|
Anthozoa
|
Subclass
|
Hexacorallia
|
Order
|
Scleractinia
|
Family
|
Pocilloporidae
|
Genus
|
Pocillopora
|
Species
|
Pocillopora acuta Lamarck, 1816
|
From:
World Register of Marine Species (WoRMS)
Synonyms
Pocillopora apiculata Ehrenberg, 1834
Pocillopora bulbosa Ehrenberg, 1834
Pocillopora caespitosa Dana, 1846
Pocillopora damicornis bulbosa Ehrenberg, 1834
Pocillopora subactua Milne Edwards, 1860
(from
http://www.marinespecies.org/aphia.php?p=taxdetails&id=759099)
Species delimitation
What defines a species, or what is considered a species is often debated among scientists and taxonomists [33]. This issue might be further complicated by the problem of a species complex, where closely related species are very similar in appearance to the point that boundaries between them are unclear. This may lead to errors in biological diversity estimates, confusing or misleading interpretations of ecological and evolutionary data [34].
The study by Schmidt-Roach et al., (2014) [6] formally revised species in the Pocillopora damicornis species complex within the genus Pocillopora into 5 different species (P. damicornis, P. acuta, P. aliciae, P. brevicornis, P. bairdi). As corals are known to display extreme phenotypic plasticity (including corals from the genus Pocillopora) [35] and/or instances of hybridization along the geneological history of the species might prevent the use of conventional species concepts [36, 37], the study used the 'Unified Species Concept' (USC) [38] in delineating valid taxonomic units.
The USC assumes species are represented by separately evolving metapopulations and are assessed based on the synergistic use of criteria associated with previously accepted species concepts. Each criterion therefore represents an independent component in a global line of evidence to support species formation [38]. The intention for the use of USC is that the debate is shifted from species definition to the reliability, relevance of particular data in delimiting species [38]. Some criticisms of this species concept include the fact that is that there is no clear definition how this 'synergistic use of criteria of previously accepted species concepts' is judged. In addition, the use of different previously accepted species concepts as criteria may result in ambiguous or conflicting results as it is possible when using the same data, to reach different conclusions of different number of species when using different species concepts [50]. This might prove to be problematic as species are thought to be complex things, with their identity dependent on the methodologies used to diagnose them and on the many different ways that organisms have of belonging to a species [49].
The USC is similar to the evolutionary species concept in that the general concept of species as separately evolving metapopulation lineages. That is the only defining (necessary) property of a species and the other properties that created incompatibilities among alternative species concepts are subject to consideration [38].
The revision was completed after consideration of all available information including those from previous studies to assess species integrity for each candidate species including molecular phylogenies, gross- and fine-scale morphology, and where obtainable or known, reproductive [39, 40] and symbiont differences [39, 41].
Symbiont differences - Does it really matter?
Considering symbiont differences is particularly interesting as there has been conflicting studies. One study found species specificity of Pocillopora coral symbiosis to algae from different clades of Symbiodinium [41]. This was contradicted by another study which showed that flexibility in associations between Pocillopora corals and Symbiodinium limit the utility of symbiosis ecology in defining species [42]. This poses an interesting question, if symbiont differences do matter, how is this different for other animals? For example if there are differences in human microbiota, does this possibly give evidence for humans with striking differences in microbiota to be separate species? As such symbiont associations should be used cautiously to assess species limits especially since they have shown to be flexible in symbiosis.
Methods of species delimitation
Molecular phylogenies were generated by MEGA4 using neighbour-joining algorithm under the JC correction for the mitochondrial ORF region with the JC correction and 1000 bootstrap pseudo-replications. Network v4.5.1.6 was also used to examine genealogical relationships in the HSP70B region using the median-joining algorithm. While gross morphology and shared morphological characteristics masked clear separation for some groups due to high levels of plasticity, fine-scale morphological variation (i.e. the shape and type of columella) differentiated between clades and proved to be useful tool in distinguishing between evolutionary relationships among genetic lineages (Figure 1)[6]. Based on a multi-level approach combining all evidence (including molecular data from mitochondria ORF region, HSP70B region, reproductive, symbiont differences) and applying species criteria in synergy as proposed by USC, the study differentiated among the species in the genus Pocillopora.
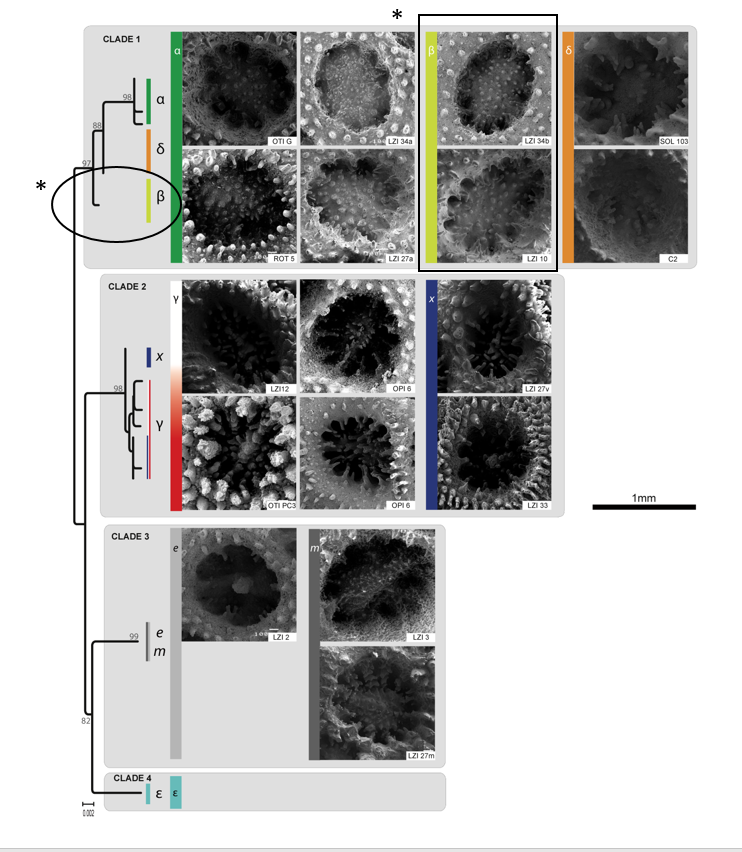 |
Figure 1: Fine-scale skeletal differences between genetic lineages with black circle and astericks indicating Pocillopora acuta. Left: phylogeny based on ORF region resolving in four main clades (bootstrap values over 70 are shown as grey numbers). Right: scanning electron micrographs of different species (bar on the left indicates scale). Clade 1: Pocillopora damicornis Type α (P. damicornis), P. damicornis Type β (P. acuta), P. damicornis Type δ (P. aliciae); Clade 2: P. damicornis Type γ (P. verrucosa), Pocillopora Type x, P. bairdisp. nov.; Clade 3: P. eydouxi e, P. meandrina m; Clade 4: P. damicornis Type ε (P. cf. brevicornis) (no micrograph available). (Source: Schmidt-Roach et al., 2014). |
Phylogeny
Higher level phylogeny: Phylum
Pocillopora acuta belongs to the class Anthozoa and subclass Hexacorallia within the phylum Cnidaria. Questions about the position of Hexacorallia and Anthozoa among the major Cnidarian taxa remain, with relationships varying depending on the use of nuclear, mitochondrial markers, and phylogenomic data (Figure 2) [43, 44, 45, 46]. The current, traditional and most widely accepted view supported by both morphological and molecular studies is that Hexacorallia is monophyletic. The dispute is mainly over the position of the tube anemones (order Ceriantharia) with a study finding Ceriantharia as sister to all other anthozoans, rendering Hexacorallia paraphyletic.
Future phylogenetic studies with increased taxonomic sampling may help to resolve more detailed relationships and patterns of character evolution in this highly diverse group [46].
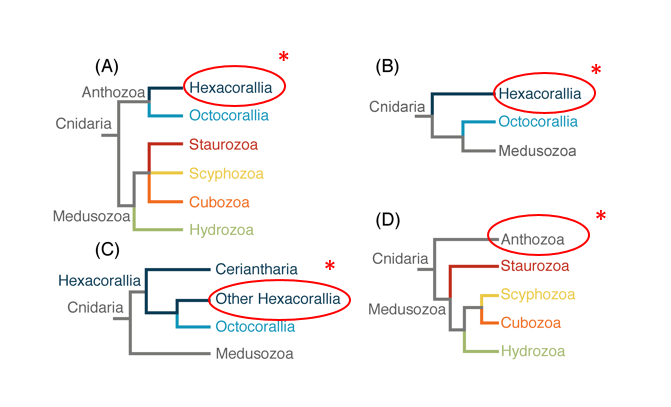 |
Figure 2. Alternative hypotheses of the cnidarian phylogeny with red circle and astericks to indicate Scleractinia position in Hexacorallia. (A) traditional view of cnidarian relationship. (B) Anthozoa paraphyletic with Octocorallia sister to Medusozoa (Kayal et al., 2013). (C) Hexacorallia paraphyletic with Ceriantharia sister to Hexacorallia + Octocorallia clade (Stampar et al., 2014). (D) Staurozoa as the sister taxon to the rest of Medusozoa (Collins et al., 2006). (Source: Zapata et al., 2015). |
Higher level phylogeny: Order
In the past, during the late nineteenth and early twentieth centuries the study of Scleractinia taxonomy and systematics relied exclusively on macromorphological skeletal characteristics. This proved problematic as hard corals including Pocillopora acuta exhibit considerable morphological plasticity, driven in part by various ecological factors [35].
In recent years, the rise of molecular tools has challenged the morphology-based taxonomy of scleractinian corals, and contributed significantly to the understanding of coral phylogenetics and evolution. Nevertheless, the coral skeleton has been and continues to be essential morphological character used in scleractinian classification.The general consensus based on current knowledge is that the order Scleractinia comprises at least 30 clades that correspond to family-level groups (Figure 3) [47]. With Pocilloporidae being in the “robust” clade. Divergence between Pocillopora and the sister genera Stylophora and Seriatopora is thought to be based on the evolution of a different reproductive mode (Spawning versus brooding in Stylophora and Seriatopora) [48].
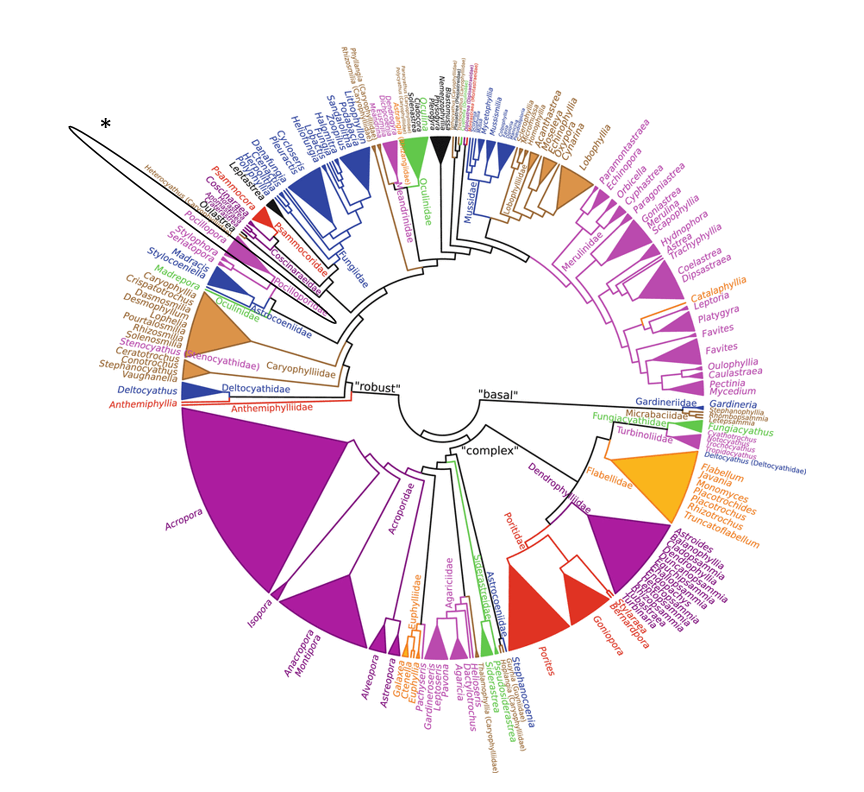 |
Figure 3: Maximum likelihood genus-level unrooted phylogeny (576 species) showing the genus Pocillopora circled in black and indicated by asterick of Scleractinia based on 12 DNA markers: mitochondrial 12S rDNA, 16S rDNA, ATP synthase subunit 6, cytochrome c oxidase subunit I, control region, cytochrome b and NADH dehydrogenase subunit 5; nuclear 18S rDNA, 28S rDNA, histone H3, internal transcribed spacers and Pax-C 46/47 intron. Branch supports not assessed in detail. Colours differentiate adjacent families and are not unique for any taxa, except for genera assigned incertae sedis that are shown in black. (Source: Kitahara et al., 2016) |
References
1. Muscatine, L., 1973. Nutrition of corals. Biology and geology of coral reefs, 2(1), pp.77-115.
2. Muscatine, L. and Porter, J.W., 1977. Reef corals: mutualistic symbioses adapted to nutrient-poor environments. Bioscience, 27(7), pp.454-460.
3. Richmond, R.H., 1987. Energetics, competency, and long-distance dispersal of planula larvae of the coral Pocillopora damicornis. Marine Biology, 93(4), pp.527-533.
4. Putnam, H.M. and Gates, R.D., 2015. Preconditioning in the reef-building coral Pocillopora damicornis and the potential for trans-generational acclimatization in coral larvae under future climate change conditions. Journal of Experimental Biology, 218(15), pp.2365-2372.
5. Ng, C.S.L., Toh, T.C. and Chou, L.M., 2016. Coral restoration in Singapore’s sediment-challenged sea. Regional Studies in Marine Science, 8, pp.422-429.
Brown, B.E., 1997. Coral bleaching: causes and consequences. Coral reefs, 16(5), pp.S129-S138.
6. Schmidt-Roach, S., Miller, K.J., Lundgren, P. and Andreakis, N., 2014. With eyes wide open: a revision of species within and closely related to the Pocillopora damicornis species complex (Scleractinia; Pocilloporidae) using morphology and genetics. Zoological Journal of the Linnean Society, 170(1), pp.1-33.
7.
Poquita-Du, R.C., Ng, C.S.L., Loo, J.B., Afiq-Rosli, L., Tay, Y.C., Todd, P.A., Chou, L.M. and Huang, D., 2017. New evidence shows that Pocillopora ‘Pocilloporadamicornis-like’corals in Singapore are actually Pocillopora acuta (Scleractinia: Pocilloporidae). Biodiversity data journal, (5).
8.
Huang, D., Tun, K.P., Chou, L.M. and Todd, P.A., 2009. An inventory of zooxanthellate scleractinian corals in Singapore, including 33 new records. Raffles Bulletin of Zoology, 22, pp.69-80.
9.
Ng, C.S.L., Chen, D. and Chou, L.M., 2012. Hard coral assemblages on seawalls in Singapore. Contributions to Marine Science, 2012, pp.75-79.
10.
Toh, K.B., Ng, C.S.L., Wu, B., Toh, T.C., Cheo, P.R., Tun, K. and Chou, L.M., 2017. Spatial variability of epibiotic assemblages on marina pontoons in Singapore. Urban Ecosystems, 20(1), pp.183-197.
11. Sorokin, Y.I., 1973. On the feeding of some scleractinian corals with bacteria and dissolved organic matter. Limnology and Oceanography, 18(3), pp.380-386.
12.
Anthony, K.R., 1999. Coral suspension feeding on fine particulate matter. Journal of Experimental Marine Biology and Ecology, 232(1), pp.85-106.
13.
Toh, T.C., Peh, J.W.K. and Chou, L.M., 2013. Heterotrophy in recruits of the scleractinian coral Pocillopora damicornis. Marine and freshwater behaviour and physiology, 46(5), pp.313-320.
14. Lewis, J.B. and Price, W.S., 1975. Feeding mechanisms and feeding strategies of Atlantic reef corals. Journal of Zoology, 176(4), pp.527-544.
15. Brown, B.E., 1997. Coral bleaching: causes and consequences. Coral reefs, 16(5), pp.S129-S138.
16.
Hoegh-Guldberg, O., 1999. Climate change, coral bleaching and the future of the world's coral reefs. Marine and freshwater research, 50(8), pp.839-866.
17. Heron, S.F., Maynard, J.A. and Ruben van Hooidonk, C., 2016. Warming trends and bleaching stress of the World’s coral reefs 1985–2012. Scientific reports, 6.
18. British Broadcasting Corporation, 2017. Great Barrier reef: two-thirds damaged in 'unprecedented' bleaching, accessed November 2017, <http://www.bbc.com/news/world-australia-39524196>
19.Jonathon Schwartz, 2017.
Trapezia cymodoce, Invertebrates of the Coral Sea, accessed November 2017, <
http://www.gbri.org.au/Classes/2012/Trapeziacymodoce%7CJonathonSchwartz.aspx>
20.
Kerr, A.M., Baird, A.H. and Hughes, T.P., 2011. Correlated evolution of sex and reproductive mode in corals (Anthozoa: Scleractinia). Proceedings of the Royal Society of London B: Biological Sciences, 278(1702), pp.75-81.
21. Harrison, P.L., 2011. Sexual reproduction of scleractinian corals. In
Coral reefs: an ecosystem in transition (pp. 59-85). Springer Netherlands.
22.
Toh, T.C., Guest, J. and Chou, L.M., 2012. Coral larval rearing in Singapore: observations on spawning timing, larval development and settlement of two common scleractinian coral species. Contributions to Marine Science. National University of Singapore, Republic of Singapore, pp.81-87.
23.
Costanza, R., de Groot, R., Sutton, P., van der Ploeg, S., Anderson, S.J., Kubiszewski, I., Farber, S. and Turner, R.K., 2014. Changes in the global value of ecosystem services. Global environmental change, 26, pp.152-158.
24. Moberg, F. and Folke, C., 1999. Ecological goods and services of coral reef ecosystems.
Ecological economics,
29(2), pp.215-233.
25. Coles, S.L. and Brown, B.E., 2003. Coral bleaching—capacity for acclimatization and adaptation. Advances in marine biology, 46, pp.183-223.
26. Graham, N.A.J. and Nash, K.L., 2013. The importance of structural complexity in coral reef ecosystems. Coral Reefs, 32(2), pp.315-326.
27.
Newell, R.C., Seiderer, L.J. and Hitchcock, D.R., 1998. The impact of dredging works in coastal waters: a review of the sensitivity to disturbance and subsequent recovery of biological resources on the sea bed. Oceanography and Marine Biology: An Annual Review, 36, pp.127-178.
28.
Rogers, C.S., 1990. Responses of coral reefs and reef organisms to sedimentation. Marine ecology progress series, pp.185-202.
29. Hilton, M.J. and Manning, S.S., 1995. Conversion of Coastal Habitats* in Singapore: Indications of Unsustainable Development. Environmental Conservation, 22(4), pp.307-322.
30. Lai, S., Loke, L.H., Hilton, M.J., Bouma, T.J. and Todd, P.A., 2015. The effects of urbanisation on coastal habitats and the potential for ecological engineering: A Singapore case study. Ocean & Coastal Management, 103, pp.78-85.
31. McCook, L.J., 1999. Macroalgae, nutrients and phase shifts on coral reefs: scientific issues and management consequences for the Great Barrier Reef. Coral reefs, 18(4), pp.357-367.
32. McManus, J.W. and Polsenberg, J.F., 2004. Coral–algal phase shifts on coral reefs: ecological and environmental aspects. Progress in Oceanography, 60(2), pp.263-279.
33.
Wheeler, Q. and Meier, R. eds., 2000. Species concepts and phylogenetic theory: a debate. Columbia University Press.
34. Bickford, D., Lohman, D.J., Sodhi, N.S., Ng, P.K., Meier, R., Winker, K., Ingram, K.K. and Das, I., 2007. Cryptic species as a window on diversity and conservation. Trends in ecology & evolution, 22(3), pp.148-155.
35. Todd, P.A., 2008. Morphological plasticity in scleractinian corals. Biological Reviews, 83(3), pp.315-337.
36. Veron, J.E.N., 1995. Corals in space and time: the biogeography and evolution of the Scleractinia. Cornell University Press.
37. Willis, B.L., van Oppen, M.J., Miller, D.J., Vollmer, S.V. and Ayre, D.J., 2006. The role of hybridization in the evolution of reef corals. Annu. Rev. Ecol. Evol. Syst., 37, pp.489-517.
38.
De Queiroz, K., 2007. Species concepts and species delimitation. Systematic biology, 56(6), pp.879-886.
39. Schmidt-Roach, S., Miller, K.J., Woolsey, E., Gerlach, G. and Baird, A.H., 2012. Broadcast spawning by Pocillopora species on the Great Barrier Reef. PLoS One, 7(12), p.e50847.
40.
Schmidt-Roach, S., Lundgren, P., Miller, K.J., Gerlach, G., Noreen, A.M. and Andreakis, N., 2013. Assessing hidden species diversity in the coral Pocillopora damicornis from Eastern Australia. Coral Reefs, 32(1), pp.161-172.
41. Pinzon, J.H. and LaJEUNESSE, T., 2011. Species delimitation of common reef corals in the genus Pocillopora using nucleotide sequence phylogenies, population genetics and symbiosis ecology. Molecular Ecology, 20(2), pp.311-325.
42. Cunning, R., Glynn, P.W. and Baker, A.C., 2013. Flexible associations between Pocillopora corals and Symbiodinium limit utility of symbiosis ecology in defining species. Coral Reefs, 32(3), pp.795-801.
43. Collins, A.G., Schuchert, P., Marques, A.C., Jankowski, T., Medina, M. and Schierwater, B., 2006. Medusozoan phylogeny and character evolution clarified by new large and small subunit rDNA data and an assessment of the utility of phylogenetic mixture models. Systematic biology, 55(1), pp.97-115.
44. Kayal, E., Roure, B., Philippe, H., Collins, A.G. and Lavrov, D.V., 2013. Cnidarian phylogenetic relationships as revealed by mitogenomics. BMC Evolutionary Biology, 13(1), p.5.
45.
Stampar, S.N., Maronna, M.M., Kitahara, M.V., Reimer, J.D. and Morandini, A.C., 2014. Fast-evolving mitochondrial DNA in Ceriantharia: a reflection of hexacorallia paraphyly?. PLoS One, 9(1), p.e86612.
46. Zapata, F., Goetz, F.E., Smith, S.A., Howison, M., Siebert, S., Church, S.H., Sanders, S.M., Ames, C.L., McFadden, C.S., France, S.C. and Daly, M., 2015. Phylogenomic analyses support traditional relationships within Cnidaria. PLoS One, 10(10), p.e0139068.
47. Kitahara, M.V., Fukami, H., Benzoni, F. and Huang, D., 2016. The new systematics of Scleractinia: integrating molecular and morphological evidence. In The Cnidaria, Past, Present and Future (pp. 41-59). Springer International Publishing.
48. Shlesinger, Y., Goulet, T.L. and Loya, Y., 1998. Reproductive patterns of scleractinian corals in the northern Red Sea. Marine Biology,
132(4), pp.691-701.
49. Agapow, P.M. and Sluys, R., 2005. The reality of taxonomic change. Trends in ecology & evolution, 20(6), pp.278-280.
50. Agapow, P.M., Bininda-Emonds*, O.R., Crandall, K.A., Gittleman, J.L., Mace, G.M., Marshall, J.C. and Purvis, A., 2004. The impact of species concept on biodiversity studies. The quarterly review of biology, 79(2), pp.161-179.